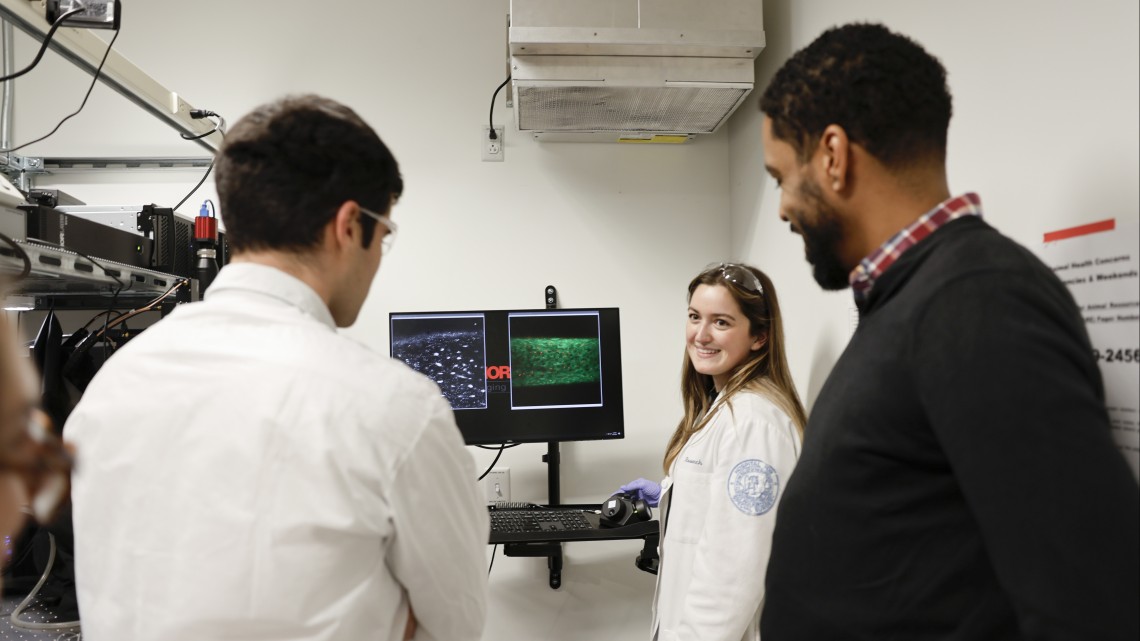
Graduate student Andi Garcia-Ortiz (middle) shows microscopy to Karl Lewis, assistant professor of biomedical engineering (right), and Alexander Saffari ’24.
News directly from Cornell's colleges and centers
Lewis Lab investigates intriguing role of osteocytes in bone health
By Jackie Swift
Osteoporosis is common in older people. In the United States, an estimated 10 million people have the disease, 80 percent of them women, according to the Bone Health and Osteoporosis Foundation. The foundation also estimates that one in two women will break a bone after the age of 50 because of osteoporosis.
Given how common this disease is, not a lot is known about the underlying bio mechanisms, says Karl J. Lewis, assistant professor of biomedical engineering at Cornell. “Osteoporosis happens to everyone if you live long enough,” he says. “But for women, there’s a rapid decline in bone mass after menopause caused by a dysregulation of the bone-resorbing cells compared to the bone-building cells. We know there’s a correlation between the big drop in estrogen leading to a large reduction in bone, but we have no idea what is changing on the cellular level.”
A special type of cell, called an osteocyte, may hold the key. Osteocytes live encased in bone material. Although they can’t move very much, they are connected to each other via dendrites, similar to the way nerve cells are connected, and they can communicate back and forth amongst themselves.
Osteocytes play a main role in building and maintaining bone, Lewis explains. They are the key cellular sensor for mechanical force, which is the primary biological input for bones. They also drive the behavior of both cells that build bone and those that absorb it. On top of all that, they mediate between bone and other tissue functions in the body. Using circulating proteins, osteocytes communicate with these other tissues, including the kidneys and gut (both of which regulate serum calcium), the brain, muscles and cartilage.
“They are a special type of cell, super fascinating and super complicated,” says Lewis. “And they’re really difficult to study because of their position in the body.”
Osteocytes: to each its own response
Lewis began his research on osteocytes as a graduate student in the Schaffler Lab at the City College of New York where he worked on a project that explored how osteocytes encode loading magnitude. Researchers already knew that higher magnitude loading yields more bone mass accrual and that osteocytes were likely responsible for sensing load. This would mean that osteocytes respond differentially to different magnitudes of force: jumping off the sixth step in a staircase would result in more osteocyte response than jumping off the bottom step, for example.
“The cells are clearly able to distinguish the difference,” says Lewis. “But we didn’t know how they were doing that. We discovered that every cell has its own threshold for responding, and increased anabolic tissue effect is a summation of more osteocytes responding to the force.”
Osteocytes that respond to a particular load can be situated next to other osteocytes that don’t respond. Not only that, but some osteocytes don’t appear to respond to loading at all. The maximum response rate is 80 to 85 percent, Lewis explains. “So what are the other 15 to 20 percent doing?” he asks.
Males versus females
From this earlier breakthrough, Lewis and his lab have continued further exploration of osteocytes. The researchers have looked at calcium signaling channels on the surfaces of the cells and investigated their impact on responses to mechanical force. They have also explored cellular components which seem to play a role in mechanical response, such as proteoglycans (which give cartilage its ability to resist compressive loads), and integrins (little hooks that cells use to latch onto their extracellular matrix).
“We’re trying to unravel the foundational information of how osteocytes mediate their mechanical environment and how that changes in a disease like osteoporosis,” Lewis says.
He and his colleagues have made a significant preliminary finding that fundamental differences in regulation of bone health exist between males and females in mouse models. “Basically, everything we’ve measured has been different in males versus females,” Lewis says. “Estrogen is such a central player for females, and although males have some estrogen, it’s nowhere near the same level. There has to be something different compensating for the role of estrogen in males.”
Among their many inquiries, the researchers have looked at the role of neuronal signaling on the parasympathetic, or rest-and-digest, side of things. “Osteocytes have receptors for acetylcholine, which is the rest-and-digest neurotransmitter,” Lewis says. “If we knock out the acetylcholine receptors in osteocytes, both males and females are affected, but the bones of males will be affected differently than the bones of females. This is one place where we reliably see sexual dimorphism.”
C’Dots reveal cell dynamics
To improve methods for studying osteocytes, the Lewis Lab has joined with Ulrich Wiesner, the Spencer T. Olin Professor of Engineering in the Department of Materials Science and Engineering, and his lab to explore the use of florescent, silica nanoparticles called Cornell Dots, or C’Dots. Developed by Wiesner, C’Dots can be injected in living organisms and targeted to specific parts of the body, such as breast cancer tumors. The nanoparticles then fluoresce under microscopic imaging, allowing surgeons to better target the tumor for removal.
Working together, the Lewis and Wiesner labs perfected a method to use C’Dots as temporary injectable fluorescent probes for use in osteocytes. Lewis wanted to use them to study matrix-binding protein dynamics in osteocytes using florescent imaging in vivo. Previous work from other groups showed that integrins may be the necessary feature that allows osteocytes to sense mechanical force, but advances since then have been limited.
“Work has been slow in the area of integrin dynamics and osteocytes because it’s such a tricky thing to study in vivo,” Lewis says. “We now have a platform for doing that, and we’re really excited about it.”
Lewis and his colleagues used the new platform to study integrin dynamics in osteocytes for the first time. So far, they’ve seen some surprising results, including apparently sexually dimorphic patterns in integrin movement to the osteocyte cell body, Lewis says. Integrins may mediate the response threshold for individual osteocytes, controlling which osteocytes respond to a given load magnitude. “The sex differences in integrin dynamics as measured with C’Dots might be a part of the differential regulation of bone mass mechanisms in males compared to females,” he says.
A born engineer
Lewis has always been intrigued by how things work. As a child, he took apart printers and watches to understand their inner workings, then tried to put them back together again. He loved cars and all their many mechanical parts. But it was only when he searched online for physics and math (his favorite subjects) together with cars that he found his calling. “Google spit back ‘mechanical engineer,’” he says. “So I did a search on mechanical engineering and learned what it was; and that’s when I found out I function like an engineer.”
Lewis studied mechanical engineering as an undergraduate but moved to biomedical engineering in graduate school when he noticed the mechanisms behind so many biological functions were still unknown. “I saw the opportunity to take my curiosity with mechanics and systems and ways to improve them and to apply that in the biomedical space,” he says. “I thought I could use my interest and expertise to understand more about certain disease states, as well as maybe create some new approaches along the way.”
Media Contact
Get Cornell news delivered right to your inbox.
Subscribe